Abstract
This paper analyzed the phenomenon of global warming, an issue that poses a serious threat to the global economy. It is a multifaceted issue that affects almost all critical sectors, including agriculture, health, and labor productivity. Global warming is the outcome of a high atmospheric concentration of greenhouse gases (GHGs), including carbon dioxide. The modern concerted international action to combat global warming and climate change is supported by years of scientific data and measurements that attribute the rising temperatures to GHG emissions from anthropogenic activities, such as fossil fuel use and destruction of forests.
Political action, which began in the 1980s, culminated in the 1994 Kyoto Protocol and the 2015 Paris Agreement to meet national GHG emission targets. The short-term impacts of climate change include health risks (high morbidity), extreme weather, and a drop in agricultural output, among others. In the long term, climate change will cause the inundation of coastal areas, leading to capital losses and mass migration. Economic tools and models used in supply-demand and cost-benefit analyses can provide a business case for concerted international action to mitigate the effects of climate change. The viable climate change adaptation and mitigation measures include carbon taxes, tradable permits, and subsidies.
Introduction
Global climate change and warming constitute the greatest environmental issue facing the world currently. Global warming is defined as the rise in mean global temperatures that is attributed to the greenhouse effect (TRS/NAS, 2014). The term ‘greenhouse effect’ denotes the atmospheric temperature levels resulting from the net retention of solar irradiation. A collection of gases termed greenhouse gases (GHGs) absorbs solar irradiation and emits some energy into space (TRS/NAS, 2014). Elevated GHG concentration in the atmosphere creates a thermal blanket that retains more solar irradiation on the Earth’s surface than it emits, leading to planetary-scale warming.
The type of global warming depends on the greenhouse gas causing the blanketing effect. The main GHGs implicated in global warming include “carbon dioxide (CO2), water vapor (H2O), methane (CH4), nitrous oxide (NO), ozone (O3), and chlorofluorocarbons (CFCs)” (Harris, Roach, & Codur, 2017, p. 4). The GHGs have different global warming potentials relative to CO2. The fundamental aspects of global warming include its causes, i.e., human influences and natural sources of GHGs (solar output and volcanicity), and effects on climate patterns. Its importance to the planet’s energy balance relates to its role in extreme weather events, sea level rise, economic impacts, etc. The development of the current global warming regime evolved from the early scientific understanding of the greenhouse effect in the early 1900s. Between 1985 and 1988, the accumulated scientific knowledge on global warming informed policy interventions. Subsequently, intergovernmental negotiations began in 1988, culminating in the 1997 Kyoto Protocol that set GHG emission limitations on nations.
Addressing global warming requires international cooperative action. Global warming is interlinked with international trade. This paper will analyze the phenomenon of global warming in the context of economic impacts and international trade. International trade creates opportunities for economic globalization for developing and developed economies through enhanced access to global markets. Suppliers in different locations can produce unique and competitive commodities for global marketplaces, taking into account the characteristics of the consumer. However, it increases the reliance on industries, contributing to high GHG emission. Therefore, international trade reflects the globalization of consumption that affects environmental sustainability through increased GHG emissions related to heavy industrialization.
This paper is organized into four major sections. The first section will focus on the evolution of the existing climate change regime. It will explore the scientific consensus on global warming leading up to policy interventions and multilateral agreements on climate change. The second section will discuss the economics of climate change. In this regard, anthropogenic causes and natural sources of GHGs, impacts of global warming in the short run and long run, and the economic impacts and implications of climate change will be analyzed. The third section will include an analysis of demand and supply side and cost and benefit analysis of global warming in the short run and long run. The final section will involve an assessment of strategies implemented to reduce the negative impact of climate change. It will involve a critical analysis of available economic policy options and mitigation strategies.
Evolution of the Current Climate Change Regime
The current understanding of climate change and its effects has its origins in the scientific consensus on the global warming problem. According to Harris et al. (2017), scientific insights into the occurrence of an ozone hole in the stratosphere in 1987 marked a major turning point in the climate change debate. Earlier environmental conservation efforts, including the creation of the Unite Nations Environmental Program (UNEP), tilted towards specific reversible pollutants, such as oil spills in oceans. The discovery of ozone layer depletion bred the current socioeconomic initiatives to tame the irreversible impacts of global warming, culminating in the 1997 Kyoto Protocol.
Scientific Consensus on Global Warming
The global warming theory gained traction in the early 1990s after scientific reports on ozone layer depletion. Earlier forums to discuss the global warming issue, including the First World Climate Conference of 1985 that brought together various governments and policy makers, did not yield much success (Weitzman, 2012). The problem of climate change was raised at related conferences held in the late 1980s. However, it was the scientific consensus of 1990 that marked the beginning of the current climate change regime. Scientific data – represented in the Keeling curve – from observatories in Hawaii had as earlier as 1960 indicated that CO2 levels in the atmosphere were rising (Weitzman, 2012). Improvements in measurements and technology in the 1970s led to the development of reliable global warming simulations by the National Academy of Sciences that laid the foundation for the scientific consensus on the greenhouse effect. In the 1980s, scientists found a link between anthropogenic GHGs such as NO and methane, painting a clear picture of the magnitude of the global warming problem.
Global warming first became a political issue in the late 1980s. Improved scientific understanding of the phenomenon spurred political interest in climate change. Weitzman (2012) notes that three other factors besides scientific consensus constituted earlier impetuses for intergovernmental action to reduce GHG emissions. First, a clique of environmental scientists endeavored to raise the profile of global warming in the international arena. These individuals worked closely with UNEP to help familiarize governments about climate change through conferences and policy papers. Later, in 1988, the Advisory Group on GHGs was set up in the UNEP, bringing together climate scientist who helped drive the climate change issue.
The second contributing factor, according to Weitzman (2012), was the growing prominence of the global issues of ozone layer depletion, water body pollutants, and species extinction. In the late 1980s, chlorofluorocarbons (CFCs) were implicated in ozone layer depletion, indicating that anthropogenic activities contribute to climate change. The third factor was the occurrence of extreme weather events, such as the 1988 heat wave that affected parts of Canada and the US (Weitzman, 2012). The drought was attributed to greenhouse warming. A conference held in Canada in the same year resolved to cut CO2 emissions by 20 percent by 2005 through a carbon tax (Weitzman, 2012). The aim was to protect the atmosphere from GHG emissions responsible for the greenhouse effect.
The Kyoto Protocol
The Kyoto protocol on climate change was a culmination of intense negotiations to cut down carbon emissions in the post-2000 period (TRS/NAS, 2014). Before 1990, the dominant players in climate change debate were nongovernmental agencies. Governments began to play an active role following the creation of the Intergovernmental Panel on Climate Change (IPCC) to lead negotiations. Binding GHG emission targets and timelines were established to reduce carbon emissions in the years to come. The negotiations for CO2 emission reductions faced obstacles from middle-income countries, such as China and Brazil, which argued for interventions that do not impinge on their sovereignty (Weitzman, 2012). On their part, oil-producing nations doubted the reliability of scientific findings. A convention prepared by the Framework Convention on Climate Change (FCCC) was ratified by fifty nations in 1994 to address ozone issues (TRS/NAS, 2014). However, the opposition of the timelines and targets by developed nations delayed the implementation of this treaty.
The FCCC process laid the foundation for intergovernmental negotiations in Berlin in 1994 that created the Kyoto protocol. It specified the obligations for developed nations to reduce GHG emissions in the post-2000 period (TRS/NAS, 2014). The major issue that dogged this protocol was the flexibility of the implementation of the emission targets. Developed countries felt that there should be flexibility in achieving the emission-limitation targets to avoid stifling economic development. Despite these challenges, the Kyoto protocol remains a useful mechanism for reducing GHG emissions. Currently, carbon taxes and sustainable development programs are useful tools for reducing the negative impact of global warming.
Economics of Climate Change
Antecedents of Global Warming and Climate Change
Since the 1800s, carbon dioxide was known to be a GHG that causes the blanketing effect. According to Weitzman (2012), atmospheric CO2 measurements indicate that CO2 levels rose by up to 40% between 1800 and 2012. A significant proportion of all CO2 emissions is attributed to human activities. Carbon dioxide balance is attained naturally through the processes of photosynthesis and respiration. Natural sources, e.g., volcanic eruptions, add an insignificant amount of CO2 (1% of anthropogenic emissions) to the atmosphere, which is balanced out by chemical processes (Weitzman, 2012). The exponential increase in CO2 emission has occurred since the 1970s, largely due to rapid industrialization.
Measurements show that increases in atmospheric CO2 are due to fossil fuel (coals, oil, and gas) combustion for hydropower and heating that emit 25 percent of GHG emissions (Weitzman, 2012). Anthropogenic emissions also result from transportation (14 percent) and farming practices – deforestation and the burning of plant material (24 percent). The carbon dioxide released from these processes affects the carbon cycle since the natural carbon sinks are fewer compared to anthropogenic emissions. The net effect is that more CO2 accumulates in the atmosphere, causing global warming. Other GHGs, including methane and nitrous oxide, also have anthropogenic origins.
The global temperature increase since the 19th century resonates with the recorded concentrations of CO2 over the same period. Climate fingerprints obtained from geographical patterns of temperature changes implicate GHG emissions. In addition, warming oceans, sea level rise, and melting of polar ice are consistent with climate change patterns occasioned by increasing atmospheric CO2 concentrations (TRS/NAS, 2014). It is clear that the amount of natural CO2 emissions is too small to have a significant impact on climate change. Further, natural sinks rapidly sequester the additional CO2 to maintain the atmospheric balance of gases.
Besides volcanic activities, the other natural causes of climate change include solar output, the Earth’s revolution around the Sun, and events like El Nino and La Nina (Harris et al., 2017). However, the heating up of the troposphere and the cooling of the stratosphere indicate that natural forces cannot singularly account for the climate change witnessed. In comparison, increases in solar output heat up both the upper and lower atmosphere. Computer models on climate change associate tropospheric CO2 increase due to anthropogenic emission with the greenhouse effect over the past four decades (TRS/NAS, 2014). Climate change – whether caused by natural factors or anthropogenic activities – has a negative impact on human societies, species, and oceans.
The extent or severity of climate change and the greenhouse effect will rely on the atmospheric CO2 accumulation and stabilization. According to the TRS/NAS (2014) report, the atmospheric CO2 concentrations in the pre-industrial era stood at about 280 ppm and increased by 60 percent between 1990 and 2014. To restore pre-industrial climate, the atmospheric CO2 levels should be maintained at 350 ppm, down from the 400 ppm reported in 2015 (TRS/NAS, 2014). If the other GHGs are considered, the greenhouse effect rises to 430 ppm, relative to CO2 (carbon dioxide equivalent or CO2e). The atmosphere has never had CO2e of this level for close to 800,000 years (TRS/NAS, 2014). The projected resultant warming effect until 2100 due to CO2 emission is illustrated in Figure 1. It shows the CO2 levels and the predicted temperature increases based on previous models. It indicates that the stabilization of GHG levels at 450 ppm will result in a global warming effect of 1.0-3.8°C.
Impacts of Global Warming and Climate Change
The increasing GHG levels in the atmosphere have had significant impacts on the planet. First, global warming is implicated in sea level rises in most parts of the world. According to Weitzman (2012), the role of global warming in the rising sea level could be attributed to two reasons. First, warmer climates occasion the melting of polar ice, adding more water into the oceans and seas. Second, the rising temperatures are warming the oceans, making them to expand. It is estimated that the global sea level increases by 3.5 millimeters annually, and by 2100, it is expected to reach 2 meters, if the current GHG emission trends continue (Weitzman, 2012).). Measurements of sea level rises between 1880 and 2012 are shown in Figure 2.
At the rate at which the sea level is rising, about two-thirds of the major metropolis worldwide will be inundated, affecting over 700 million people (Harris et al., 2017). Currently, about eleven islands, forming part of the Solomon Islands, face imminent inundation. Conservative estimations indicate that increasing sea levels will force 0.66-1.7 million people living in the Pacific areas to seek shelter elsewhere by 2050 (Harris et al., 2017). The inundation of coastal areas comes with huge economic costs. In the U.S., sea level increase will lead to losses amounting $238-507 billion of investments in metropolises like Miami, unless timely interventions are made (Harris et al., 2017).
In the short run, the most significant impact of global warming is extreme weather events. A warmer troposphere is more humid due to global warming, which results in heavy precipitation in some areas and drier weather in others (Weitzman, 2012). Intense and extreme weather is becoming more prevalent in regions that previously experienced moderate climates. In addition, rainfall patterns are changing globally, resulting in extreme droughts and famines. Areas in the American West and countries in the Horn of Africa, including Somalia, have experienced severe droughts in since 2010 (Weitzman, 2012). Extreme weather events, such as hurricanes, have become more intense in the U.S., Latin America, and Pacific Islands, e.g., the Philippines, killing thousands of people and displacing millions.
Global warming will also impact food production negatively in the short run. By 2014, irrigation agriculture constituted 16 percent of the farmland, contributing 36 percent of food production globally (Harris et al., 2017). Warmer climates will lead to erratic rainfalls, leading to increased dependence on irrigation agriculture. In the next decade, the demand for water will increase exponentially due to widespread desertification. Going by the GHG emission projections, the number of arid areas will grow to 30 percent, up from the current 1-3 percent by 2090 (Harris et al., 2017). From these statistics, it is clear that the global food production is likely to go down. Warmer climates may enhance growth and lead to higher yields in colder areas. However, warmer conditions are ideal for pests and weeds, which affect food production. In addition, increased salinity in coastal areas due to sea level rises will lead to poor yields.
Another immediate impact of global warming relates to political risks. Climate change leads to higher food prices, which create civil unrest in most countries. Food riots that turn tragic have been seen in countries prone to extreme weather events, such as Haiti (Harris et al., 2017). Global warming exacerbates famine, which breeds resource conflicts between communities and countries. Climate change also comes with certain human health risks, including heat waves. Disease vectors and insects that thrive in warmer weather are likely to increase the incidence of infections in the population. The accumulation of air pollutants, such as smog, due to a warmer troposphere and coal combustion will increase the incidence of respiratory complications and deaths.
The combustion of fossil fuels in industries causes an estimated 24,000 fatalities yearly attributed to respiratory complications, costing the economy $187.5 billion annually in health care costs and absenteeism (Harris et al., 2017). Climate change also affects ecosystems negatively. Many species are endangered and at risk of extinction due to habitat loss or droughts. It is predicted that up to 50 percent of the planet’s terrestrial and pelagic species will become extinct due to rising ocean temperatures. Moreover, increased ocean acidity due to high atmospheric CO2 concentration has occasioned the disappearance of coral reefs that are sensitive to pH changes.
Economic Impacts and Implications of Climate Change
The economic costs of global warming are based on climate change models that arose in the mid-1990s. These models integrate biophysical variables with economic models to estimate the socioeconomic impacts of GHG emissions and climate change (Weitzman, 2012). An example is the ENV-Linkages model adopted by the OECD to quantify climate change effects. It examines clime change impacts on various sectors, including agriculture, health care, energy, etc. The ENV-Linkage model predicts that these effects will intensify at a higher rate than the global Gross Domestic Product in the long run.
In the absence of policy responses, the net effect of the impacts on the world GDP will reach 1.0-3.3 percent by 2060 (Harris et al., 2017). These data are based on the projected twofold increase in atmospheric CO2, causing global temperatures to ranges of 1.5-4.5oC. However, according to Harris et al. (2017), if the temperature range reaches 1.0-6.0oC, the global economy will shed 0.6-4.4 percent of annual GDP. Further, if the planet warms to 4oC above the pre-industrial estimates in the next century, the global GDP losses will reach 1.0-10 percent. The statistics suggest that continued GHG emission will result in significant economic damages in the long run.
In particular, the global agricultural output will be affected by climate change by 2015. Based on this model, region-specific and sector-specific estimates indicate that global warming will affect the agricultural output of crops such as rice, wheat, oil seeds, fruits, and vegetables in OECD regions, Latin America, Asia, and Sub-Saharan Africa (Weitzman, 2012). For example, grain output per hectare is projected to decline by over 45 percent in Indonesia by 2050 due to droughts. From the ENV-Linkages model projections, declines in rice production by 2050 are highest in tropical regions of Africa, Latin America, and Asia (Harris et al., 2017). However, certain countries, e.g., Chile, will experience increased rice output by 2050. Further, areas with colder climates, e.g., Scandinavia, will experience improved agricultural output due to global warming. It is evident that global warming will affect some regions positively and others negatively. Therefore, the international trade patterns for crops like rice are likely to shift by 2050.
Global warming will also impact on the global fish output, hurting the economy of countries with a thriving fisheries industry. Extreme conditions, including warming oceans, acidification, and salinity, will reduce fish habitats and reduce the total catch. Based on the ENV-Linkages model, fish stocks will decline significantly in North Africa by 27 percent and Indonesia by 26 percent by 2050 (Harris et al., 2017). Regions in Europe and Far East will experience moderate impacts of -10 to -15 percent, while Latin America will be affected marginally. Fish catches will improve in Russia (+25 percent) and other countries in Europe.
Another economic impact of climate change results from coastal land losses. Rises in sea levels will affect physical capital investments in coastal zones, which will hurt national economies. Coastal cities risk complete inundation due to rising sea levels. The estimated cost related to property damage in US coastal cities stand at $238-507 by 2050, if adaptation measures are not undertaken (OECD, 2015). The effects are quantified in terms of land losses (in square kilometers) and capital losses. The coastal areas most vulnerable to inundation are located in South East Asia. According to Weitzman (2012), it is projected that by 2050, land and property losses will amount to -0.60 percent for the Indian economy based on the 2000 baseline. The other developing countries of Asia will incur combined losses of -0.86 percent. Regions in North America will experience marginal land losses with the most affected being Canada (-0.46 percent).
The negative economic impacts have increased due to extreme weather events. Costs related to property damages from extreme events, such as hurricanes, are increasing. Intense storms that are predicted to increase in frequency this century will decrease capital stocks in all sectors. Tropical cyclones alone cause global losses amounting to $26 billion or 0.04 percent of world GDP yearly (Weitzman, 2012). The economic losses are projected to double, as cyclones will become more frequent and intense in the coming years because of climate change. The most affected regions by cyclones will be North America, the Pacific Island, and parts of Asia.
Another critical sector of the global economy that is vulnerable to climate change effects is public health. The health impacts of climate change encompass the direct effects of heat waves and vector-borne diseases. As a result, mortality and morbidity due to climate-related conditions will increase as the planet becomes warmer. Infectious illnesses, such as malaria, cardiovascular complications, and respiratory disorders are on the rise due to warmer conditions that are ideal for vectors to thrive (OECD, 2015).
From the labor productivity perspective, the global economy will suffer due to costs related to a high disease incidence in the population, premature deaths, and occupational heat stress. Reductions in labor productivity resulting from the high morbidity rate will reduce the global output. The greatest impact will be felt in Africa, where labor productivity will decline by 0.6 percent in South Africa and 0.5 percent in the MENA region by 2050 (Weitzman, 2012). The most affected sectors are agriculture and the manufacturing industry. Other regions affected include Asia (-0.3 percent) and South America (-0.2 percent). However, in some regions, e.g., Russia, labor productivity is expected to rise by up to 0.5 percent by 2050. A second dimension of health impacts of climate change is health care spending. Treatment costs for vector-borne illnesses are projected to increase by 2060. The health care spending (as a percentage of GDP) will be highest in Asia at 0.5 percent and the MENA region at 0.3 percent (Weitzman, 2012). Interestingly, the projected demand for health services is negative in European countries like France at minus 0.1 percent.
Climate change has also disrupted the dynamics of energy demand and supply globally. The GHG emission cuts and limitations coupled with rising temperatures impact the residential demand for electricity and gas. Global warming will raise the demand for cooling and heating in residential and business premises. According to the OECD (2015) report, the global energy demand based on the World Energy Model is projected to “grow by 250 percent between 2010 and 2060” at the current global warming levels and by 330 percent if the climate change trend continues (p. 12). The energy demand will be highest in non-OECD economies. By 2050, the energy demand for cooling will increase to 27 percent, up from 9.0 percent in 2010 (OECD, 2015). Most of this energy will go into air conditioning and heating due to hotter summers and colder winters, respectively. The changes in energy supply and demand will lead to high oil prices that will hurt the global economy.
Tourism is another sector of the global economy currently feeling the effects of climate change. The number of tourist destinations is expected to drop, affecting the economies that depend on tourists. Receding snow could reduce the number of skiers visiting in the Alps (OECD, 2015). Thus, the attractiveness of the leading tourist destinations will decline due to global warming. As a result, the per capita income of such economies will decline. The decline in the number of destinations will raise the price of tourism services, affecting the consumption patterns (OECD, 2015). In effect, the dwindling global spending on tourism due to climate-related pressures will hurt the global economy. However, the effects on the economy will vary according to regions. Some countries will be affected by climate-induced destruction of tourist destinations, while others will gain from the opening up of new destinations. Based on the ENV-Linkages model, by 2060, the economies projected to gain most from tourism include “Canada (+92 percent), Russia (+66 percent), and the United States (+21 percent)” (Harris et al., 2017, p. 16). The highest negative impacts on tourism will occur in Brazil (-27 percent) and Asia (-20 percent), among other countries.
Demand and Supply Side Analysis and Cost and Benefit Analysis
Demand and Supply Side Analysis
As seen from the previous section, climate change has had a net negative effect on the global GDP. It causes reductions in capital stocks and business disruptions, which hurts the world economy. Fankhauser and Stern (2016) use the global production function (Figure 3) to illustrate the impact of the changing climate on output. From the illustration, climate-related damage may cause a decline in the global capital stock, which impacts labor productivity negatively, resulting in a drop in global output. Other factors contributing to lower output include food insecurity and higher morbidity rates related to increased prevalence of communicable diseases. These variables may lead to civil unrest and labor availability and effectiveness to maintain a higher global output (Fankhauser & Stern, 2016). These dynamics can be illustrated by a demand and supply curve, as shown in Figure 4. It shows that climate change will constrain supply at any demand level. As a result, the supply curve will shift from S1 to S2 (supply decline). The outcomes of the contracted supply will be diminished output (Y2) and higher prices (P2).
The analysis in Figure 4 follows the ceteris paribus perspective, which holds that “the global population does not respond to climate change” (Fankhauser & Stern, 2016, p. 97). However, it is possible that specific measures will be adopted to mitigate the effects of global warming. In the long term, the mitigation measures could lower the economic effects of climate change. However, in the short run, such actions may involve higher economic costs by redirecting resources meant for other sectors to climate change mitigation. According to the OECD (2015) report, the greatest threat of global warming to the global economy relates to the inadequate and inefficient mitigation measures undertaken by governments. Climate change adaptation is likely to involve high economic costs, estimated to reach 1.0 percent of the world GDP yearly by 2050 (OECD, 2015).
A key supply-side variable is agricultural output. Outputs from sectors, such as agriculture, will decrease, resulting in higher prices (demand). The net effect of this trend on the world economy is inflation. Extreme weather events, including droughts, will decrease crop yields. A lower supply of commodities will mean higher food prices globally. As a result, consumers will have to spend more on food, resulting in food-related inflationary pressures. However, it is important to note that the negative impacts of droughts will be offset by increased output in other areas. Higher temperatures may cause massive migration to the more habitable regions of the world. These movements will have significant implications on regional socioeconomic dynamics and growth.
Higher energy costs will be another source of inflationary pressures. As aforementioned, climate change will result in unusually hotter summers and colder winters, boosting the global energy demands (OECD, 2015). The energy supply may not match up with the demand because the hotter climate will reduce the electric power generation efficiency (OECD, 2015). The current policy interventions are geared towards promoting renewable energy sources. The transition to renewable energy is projected to drive inflation in the short run, as governments increase investments in this Subsector. However, a greater reliance on cheaper clean energy sources will reduce energy costs and mitigate the effects of climate change in the long run.
Shifts in the supply-demand curve may also be attributed to insurance costs. The effects of climate change, including extreme events, have negatively affected the insurance industry. Damages from hurricanes and floods have occasioned massive payouts to affected businesses and families. For example, in 2011, the insurance sector paid out over $126 billion to businesses due to weather-related damage (Fankhauser & Stern, 2016). The sector is likely to respond to the changing and unpredictable climate by raising premiums. Higher insurance costs will affect supply, as most firms will decline to provide cover due to the climate risk.
Cost and Benefit Analysis
A cost-benefit analysis of global warming helps inform policy actions. The projected GHG emission by 2040 (Figure 5) in the absence of mitigation measures are worrisome. Cost-effective policies are needed to stabilize and cut down GHG emissions as the century progresses. A cost-benefit analysis involves a comparison of the costs of the policy actions agreed upon in the 2015 Paris Agreement against the impacts of the projected GHG emissions in the long term (Harris et al., 2017). Additionally, the benefits of such adaptation policies (avoided costs) are weighed against projected implementation costs. The cost-benefit analysis captures the climate-related impacts on economic production in areas such as agriculture, fisheries, and real estate.
Damage estimates are central to the cost-benefit analysis. Based on the projected magnitude and frequency of catastrophic events, e.g., hurricanes, the economic cost of climate change can be predicted. For instance, the estimated damages from the 2005 Hurricane Katrina stood at $100 billion and more than 1800 deaths (Fankhauser & Stern, 2016). If the weather events are more frequent and intense, then the estimates of damage costs will rise. In addition to hurricane frequency, increases in human morbidity and mortality, declines in agricultural output, and sea level rises due to global temperature rise have been captured in integrated assessment models as damage functions. The economic costs are expressed as a percentage of the global GDP (Figure 6). The available models shown in Figure 6 give damage estimates of between 2 percent to 10 percent of world GDP annually.
The monetary estimates of climate-related costs do not include all impacts of climate change. However, they give a rough estimate of the costs. A cost-benefit analysis also evaluates the benefits of mitigation measures against the implementation costs. Models that estimate the costs of policy actions related to climate change weigh inputs, e.g., labor, against outputs (Fankhauser & Stern, 2016). To reduce GHG emissions, fossil fuels must be replaced with clean and efficient renewable energy sources. This transition would require heavy investments in renewable energy technologies. Economists suggest that the transition will have a negative effect on GDP due to heavy technology investment and inflexible economic models. For instance, it is estimated that the implementation of the Paris Agreement goal of <2oC global warming effect would cost over 1.5 percent of the global GDP (Fankhauser & Stern, 2016). This conservative estimate is based on the assumption that all countries will cooperate. However, if there is no cooperation, the costs are projected to increase to as high as 4 percent of the world income.
Another aspect of the cost-benefit analysis is related to future costs and benefits. The valuation of the future damages of climate change or benefits of policy actions involves a discount rate. Economic models based on this assumption predict that climate change will cost the global economy 5 percent of GDP annually (OECD, 2015). On the other hand, the annual cost of policy actions aimed at cutting down GHG emissions will be about 1 percent of the world GDP. From these estimates, the cost-benefit ratio of 1:5 is obtained, which favors prompt policy initiatives to reduce CO2 emission. The net present value (PV) of future damages or gains relies on discounting. A large discount rate will result in fewer benefits and higher costs in the short run. In comparison, a low discount rate results in a greater valuation of benefits and lower costs in the long run, as shown in Figure 7. Economic models also consider the regional inequalities in global warming impacts.
Strategies Implemented to Reduce the Negative Impacts of Climate Change
Strategies for combating climate change fall into two major classes, namely, adaptive responses and preventive actions. Adaptive actions are meant to address climate change impacts, while mitigation measures aim to reduce the intensity or severity of global warming (OECD, 2015). Examples of adaptive responses include dike construction to contain sea level rises and prevent inundation, adoption of innovative agricultural practices, and resource mobilization towards humanitarian and disaster responses. In contrast, mitigation measures include cutting down GHG emission by using renewable energy alternatives, improved energy efficiency, and expanding natural carbon sinks through forestation.
In deciding on the most economically viable adaptive or mitigation measures to adopt into policy, a cost-effectiveness analysis of the available options is required. This market-oriented approach considers societal goals in evaluating policy options. Market-oriented approaches involve incentives for corporations and industries to cut GHG emissions, as opposed to punitive measures to control pollution (Weitzman, 2012). They include pollution taxes and incentives for a greater use of clean energy. Overall, mitigation measures go hand in hand with adaptation interventions because reversing the effects of climate change to attain the pre-industrial atmospheric GHG levels is not possible. The specific sectors that require adaptation measures based on the IPCC recommendations are agriculture, population health, and water (Weitzman, 2012). Precipitation patterns and vector-borne disease prevalence will vary between regions with the developing nations being the most affected. The economic policy options for adoption and mitigation of climate change are discussed below.
Carbon Taxes
The main cause of global warming is carbon emissions from fossil fuel combustion. Carbon taxes are levies imposed on firms that use fossil fuels. The tax is proportional to the estimated carbon emitted from the processing and use of oil, coal, or gas. Its goal is to increase the cost of fossil fuels and force users to adopt energy efficient technologies or move to cleaner energy sources that attract lower taxes (Weitzman, 2012). The amount of tax levied depends on the estimated social cost (monetary impact) of GHG emissions, which stands at $11-212 (Weitzman, 2012). The wide variation in the social cost is attributed to different discount rates and uncertainty estimations.
Carbon taxes affect the cost of fossil fuels, including coal. The tax (expressed in dollars per ton of carbon dioxide emitted) and its estimated impact on coal price are given in Table 1. The tax level is proportional to the carbon content of each fossil fuel. Coal, which has the highest carbon content, attracts the heaviest tax. In contrast, natural gas attracts the lowest tax because of its low carbon content (Figure 8). The demand for fossil fuel is negatively correlated with price spikes. The demand is likely to reduce when prices increase due to carbon taxes. In the short run (< 1 year), the demand elasticity due to the carbon tax is estimated to range between -0.03 and -0.25 (OECD, 2015). In this regard, a 10 percent rise in fossil fuel price would result in a short-term demand decline of between -0.30 and -2.5 percent. However, in the long run (> 5years), the expected demand elasticity decline is -0.64, which will vary across countries or regions.
Tradable Permits
Another economic strategy for reducing carbon emissions is through tradable carbon permits. In this approach, which is also known as the cap-and-trade scheme, involves allocating each company or industry the maximum carbon emission limit (Harris et al., 2017). The aggregate carbon permits would constitute the country’s GHG target. The other alternative is to award permits to GHG emission sources, including automobiles, fuel producers, and power companies. The third option entails awarding permits to the highest bidders to raise revenue for climate mitigation and adaptation. It can also involve inter-firm trade permits, whereby companies with low emission levels receive permits that they sell to those penalized for high emissions. Another strategy entails awarding carbon credits to nations that meet the GHG emission targets or energy efficiency goals. Heavy polluters can be made to pay carbon credits to nations investing in emission reduction measures like forest expansion (natural carbon sinks).
In practice, tradable permits are good incentives for adopting low-cost carbon reduction strategies. Firms will most likely adopt low-cost emission-reduction methods that will not hurt their profitability. Such schemes have been used to lower sulfur and NO emissions with a significant level of success (Harris et al., 2017). In addition, developing nations may also sell carbon credits obtained from forest expansion to industrialized countries with high emission levels. In this case, the permit price will depend on the willingness of heavy polluters to buy them. The main advantage of tradable permits is that it has a direct impact on emission reduction. In contrast, carbon taxes reduce emissions indirectly through penalties; hence, it is prone to political resistance.
Alternative Policy Options
Alternative policy measures can be adopted in cases where carbon taxes and tradable permit systems are not feasible. One such policy is the shift from fossil fuels to renewable energy. According to Fankhauser and Stern (2016), a good number of nations have invested or introduced subsidies for renewable energy to meet their carbon-emission targets. The subsidies are meant to increase investments in clean energy, including solar, wind, etc. The second policy alternative entails investment in energy efficient technologies. It encompasses regulatory requirements for the utilization of fuel-efficient machines and appliances or low-carbon sources in homes and industries. Such regulations will tilt energy use behavior in favor of renewable sources.
Research and development (R&D) investments in cleaner energy is another strategy for combating the effects of climate change. Direct investments in R&D programs will help accelerate the development of alternative energy sources. In particular, the development of non-carbon technologies, such as electric car engines, will lower the costs associated with carbon taxes (Weitzman, 2012). Such an approach will also reduce the need for carbon taxes. Reductions in carbon emissions could also involve technology transfer to industrializing nations. These countries rely heavily on fossil fuels to drive their development projects, which are mostly funded by the World Bank and IMF. Directing the foreign aid and loans towards cheaper renewable energy sources will force the developing world to shift to non-carbon energy sources.
Summary and Conclusions
From the analysis, it is clear that the phenomenon of climate change is a global problem with immense implications for agriculture, human health, political stability, and energy utilization. It results in wide temperature variations, extreme weather events, erratic precipitation, and sea level rises that threaten human habitats and the global economy. High GHG concentrations in the atmosphere cause a greenhouse effect, which is implicated in global warming. Carbon emissions from anthropogenic activities, such as fossil fuel burning, and natural processes, e.g., volcanic eruptions, increase the atmospheric levels of carbon dioxide, methane, and other greenhouse gases that entrap solar irradiation.
The impacts of climate change are many and varied. One long-term biophysical impact includes sea level rise that threatens livelihoods in coastal cities and capital investments in these areas in the coming years. In the short run, climate change has been associated with frequent and severe hurricanes and cyclones, intense droughts that affect agricultural output, political unrest, species extinctions, and health risks. The effects of these biophysical changes are being felt on the global economy. The rising temperatures are projected to reduce the global GDP by up to 4.4 percent by 2050, based on the ENV-Linkages model. The most affected sectors include crop farming, fishing, tourism, and public health.
Economic tools are useful in quantifying the effects of climate change and the cost-effectiveness of responses. A demand and supply analysis reveals that climate change will cause a decline in the annual global output (supply) due to low labor productivity that is linked to climate-related morbidity, costly climate adaptation measures, and poor crop yields. The demand will rise due to costly food prices, high insurance costs, and increased consumption of health services, among others. In selecting optimal policy responses, the cost-benefit analysis is critical. It reveals that immediate and effective adaptation and mitigation measures can help contain the effects of high atmospheric GHG concentration and curb future emissions. Viable policy actions such as carbon taxes, government subsidies, and tradable permits promise different levels of success. Overall, tradable permits appear more cost-effective than carbon taxes that tend to be punitive. The adoption of renewable energy coupled with energy efficiency measures can help cut carbon emissions and combat climate change.
References
Fankhauser, S., & Stern, N. (2016). Climate change, development, poverty, and economics. In K. Basu, D. Rosenblatt & C. Sepulveda (Eds.), The State of Economics, the State of the World (85-105). Cambridge, MA: MIT Press.
Harris, J. M., Roach, B., & Codur, A. (2017). The economics of global climate change. Medford, MA: Global Development and Environment Institute.
OECD. (2015). The economic consequences of climate change. Paris, France: OECD Publishing.
The Royal Society/National Academy of Science [TRS/NAS]. (2014). Climate change: Evidence and causes. Web.
Weitzman, M. (2012). GHG targets as insurance against catastrophic climate damages. Journal of Public Economic Theory, 14(2), 221–244. Web.
Appendices
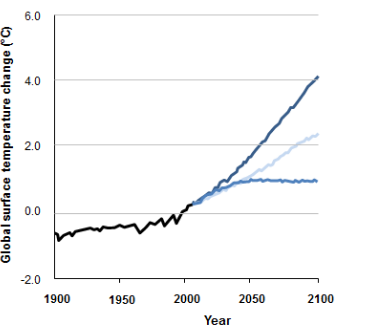
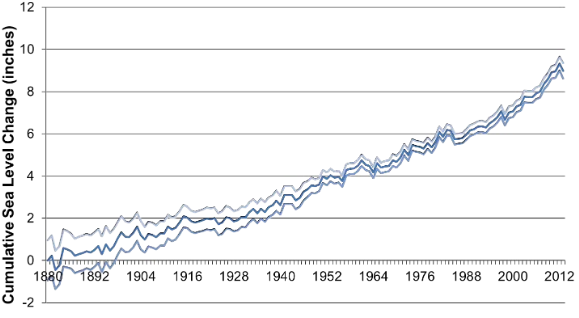
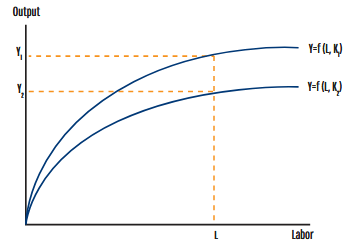
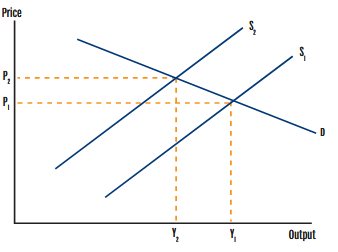
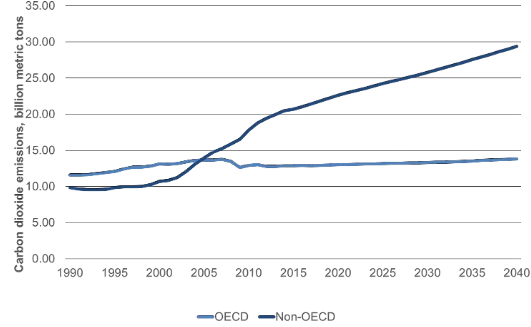
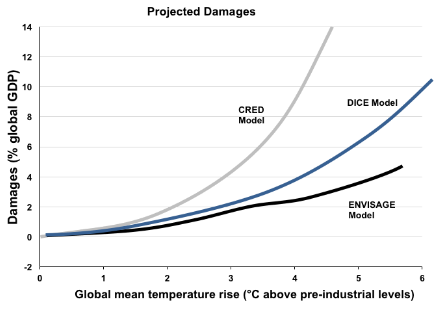
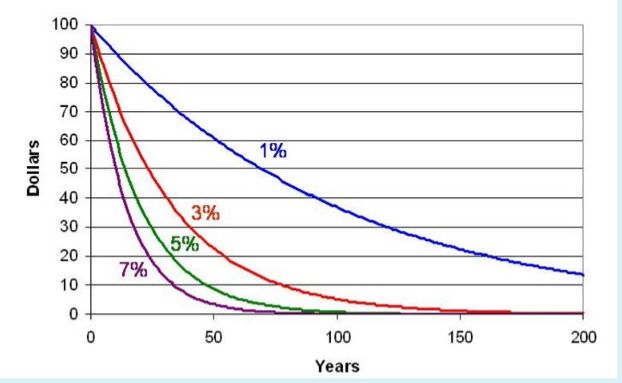
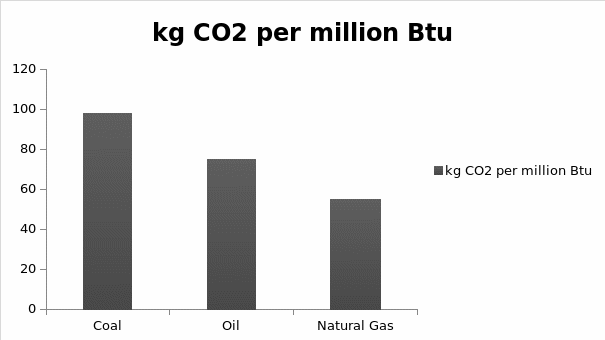
Table 1: Impact of Carbon Price on Coal Price. Source: Harris et al., 2017.